Corals at the University of Washington
The Laboratory for Engineered Materials and Structures (LEMS) is located in the Department of Aeronautics and Astronautics at the University of Washington. Like most of our NSF team members, we are also new to corals. However, we – as aerospace engineers – hope to bring new perspectives to coral research1. Specifically, we plan to apply advanced materials science and mechanics techniques to coral research. As an initial step, we focus on the following three tasks: (1) observation of coral growth using digital image correlation (DIC) and other advanced imaging techniques; (2) validation of mechanical vibration effect on the coral growth; and (3) computational fluid dynamics simulation of microfluidic devices for coral-on-a-chip studies.
1. Observation of coral growth
A. Static growth
Corals grow very slowly, with growth rates of 0.3 to 2 centimeters per year for massive corals, and up to 10 centimeters per year for branching corals [1]. Such subtle growth can hardly be detected in a short time frame, e.g., days or even weeks of time. In our group, we plan to use two methods to capture how corals grow. One is the change detection algorithm, which has wide applications in the remote sensing to detect change between two images [2]. Another is the digital image correlation technique, which has been widely used to measure the subtle deformation in materials and structures in the experimental mechanics field [3].
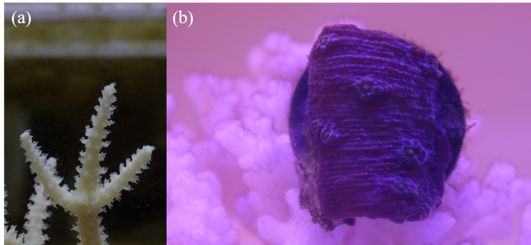
Figure 1: The images of Acropora and Montipora.
To apply these techniques, we first need to acquire coral images. We implemented two experiment setups in different labs. One is in Prof. Alex Gagnon's lab2 to observe the Acropora growth [Fig. 1(a)]. The other one is in LEMS (our lab) to observe the Montipora growth [Fig. 1(b)]. In these labs, we set up the cameras which take digital images of corals every 2 to 10 minutes. We have been observing the Acropora for almost two months, but unfortunately, the branches that we have selected showed unusually impeded growth. We are currently applying the image processing techniques mentioned above to catch such subtle growth. The Montipora has shown a very sensitive response to light conditions so far, and we are analyzing such behavior now.
B. Dynamic motions
While the growth of the coral skeleton is a very slow event, corals also show dynamic behavior due to their polyp motions. Some corals have regular pulsations, which can help with the photosynthesis. Studying dynamic motion of coral can help us know the health condition of corals, which may be affected by temperature, PH and so on. We study the motion of the polyps of Acropora by post-processing the change map and DIC results. Figure 2(a) shows the percentage of the change part in raw images as a function of time. This change part is due mostly to the polyp motions in Acropora, which has been quantified by our change detection algorithm. There is a clear decrease of the dynamic change trend around 25th day and 40th day of the measurement. The spectrogram is also used to analyze the frequency change of this signal, which is shown in Figure 2(b). The clear frequency decrease can be seen around 25th day and 40th day. Part of the reason is that the pump was turned off accidentally during this period.
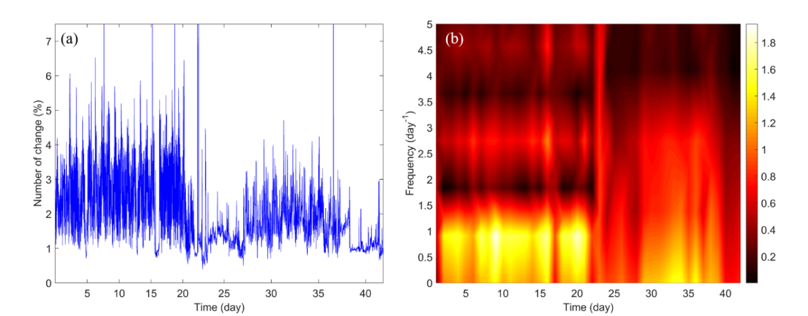
Figure 2: (a) The percentage of the number of change part as a function of time. (b) The spectrogram of the signal.
2. Effect of vibration to the coral growth
The effect of vibrations is rarely explored in marine biology field. However, sonic-assisted bone healing in human is a well-known phenomenon. Animal and human studies suggest that high-frequency and low-magnitude vibration therapy improves bone strength by increasing bone formation and decreasing bone resorption [4]. Our fundamental question is: Can vibration facilitate the coral growth? No literature has reported this mechanical vibration effect, though one study reported that pulsation can enhance the photosynthesis of the coral [5]. In this project, we plan to fabricate and test the scaffold with embedded piezoelectric materials (see Figure 3 for a 3D-printed prototype). We will also accompany this experimental work with computational fluid dynamics (CFD) simulations.

Figure 3: The image of the vibrating scaffold prototype.
3. Design of the microfluidic device for coral
Microfluidic device is a useful platform to observe the coral growth in the very early stage and to carry out well-controlled experiments to study the coral toxicology. Micro-scale interactions between corals and their environment can provide useful information about coral behavior. Coral-on-a-chip, combining micropropagation and microfluidics, makes it possible to allow direct microscopic study of live coral polyps [6]. A proper design of microfluidic device can allow us to better study coral calcification, coral–pathogen interaction and the loss of algal symbionts (coral bleaching). We are assisting Lewinski group to design the microfluidic device by conducting the CFD simulation. Based on the simulation results, optimal microfluidic device will be designed.
4. Future work and goals
We are still in a very early stage of coral research. We will continue collecting coral images and processing them via various techniques, including – but not limited to – the change detection algorithm and the digital image correlation method. For the vibrating scaffold, we will try to implement the prototype in our water tank and will start testing the vibration effect on the growth of corals. Lastly, we will explore various designs of microfluidic channels and will narrow down the design options in collaboration with Lewinski and other teams.
References:
[1] Robert D. Barnes, Invertebrate Zoology, Fifth Edit (Harcourt Brace Jovanovich College Publishers, 1987).
[2] T. Celik, IEEE Geosci. Remote Sens. Lett. 6 , 772 (2009).
[3] T. C. Chu, W. F. Ranson, and M. A. Sutton, Exp. Mech. 25 , 232 (1985).
[4] W. R. Thompson, S. S. Yen, and J. Rubin, Curr. Opin. Endocrinol. Diabetes. Obes. 21 , 447 (2014).
[5] M. Kremien, U. Shavit, T. Mass, and A. Genin, Proc. Natl. Acad. Sci. 110 , 8978 (2013).
[6] O. H. Shapiro, E. Kramarsky-Winter, A. R. Gavish, R. Stocker, and A. Vardi, Nat. Commun. 7 , 10860 (2016).
-
Our story on the initiation of this NSF:HDR research has been featured by UW media: https://www.washington.edu/news/2019/11/15/uw-aerospace-engineer-part-of-coral-grant/ ↩