How does your (coral) garden grow?
Reading our previous blog posts you will see that we keep corals in the various labs contributing to the project. Coral husbandry is very common in marine sciences (Fig. 1), it allows us to study coral colonies under controlled conditions and assess their behaviour, biology and physiology. We can also perform exposure experiments where one particular parameter is modified or a particular substance is added to the seawater to test the response of the corals. This method is very informative but has some limitations linked to the complexity of the organisms we are dealing with. Indeed, many species of coral form colonies with complex interconnections between polyps (individual corals, Fig. 2). Furthermore, the polyps live in symbiosis with dinoflagellate algae in their tissue and support a well developed microbiome that also benefits from mutualism. It is hard to clearly make sense of all biophysical implications experiments have on these systems and how one influences the other.
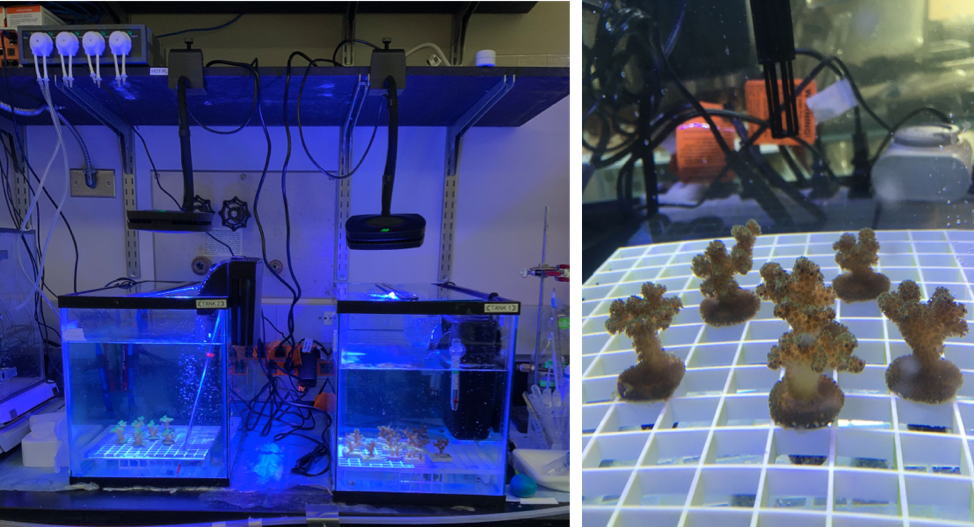
Figure 1: The tank setup in the Lewinski lab at VCU (Source: Evan Lawrence, Lewinski Lab, VCU).
One of the objectives of this project is to design experimental chambers that can help better understand and study these reactions and relationships at different scales, from the colony level (polyp-to-polyp interactions) to the cell level (cell-to-cell interactions). Before testing such experimental chambers, the coral culture method as a whole needs to be streamlined. To this effect, our group has focused on culture protocol development and troubleshooting. Each step of the culture protocol has been carefully studied and previously reported methods tested to quantify success through yield and cell survival for systematic comparison.
Methods tested:
- Harvesting and isolation - mechanical detachment of tissue (scrapping, brushing, water pik, air brush) and cells vs. chemical detachment (enzyme digestion of cell-to-cell bonds: trypsin and lyberase);
- Growth media composition and antibiotic treatment - different growth cocktails were tested using seawater supplemented with FBS with DMEM or RPMI and antibiotics-antimycotics + gentamicin solution (different concentrations of each reagents);
- Cell sorting: density gradient (Percoll) vs. fluorescence activated cell sorting (FACS) vs. microfluidic device for sorting;
- Cell attachment - plain glass vs. tissue culture treated plastic vs. Primera vs. 3D-printed PLA vs. coated plastic;
The composition of the growth medium had to be carefully adjusted to provide enough food for the cells to grow while simultaneously preventing microbial overgrowth (Fig. 3). The growth medium described briefly above does not take into account the specific needs of the different cell types. A few studies have tried to inventory the different cell types that make up corals based on their functions and returned different numbers each time. That being said, certain cell types are consistent across studies: nematocysts (stinging cells), desmocytes (cells that anchor the tissue to the skeleton), mucocytes (mucus production), calicoblastic cells (skeleton production), symbiotic cells (host the dinoflagellate algae), fibroblasts (structural scaffold), neurons (nerve cells, information transmission), epitheliomuscular cells (tissue movements) and ciliated support cells (support), pigment cells (colour, UV protection). Since these cells fulfill different functions they most likely have different needs. For example, symbiotic cells produce glucose through photosynthesis therefore might not need as much added glucose as other asymbiotic cells that make up coral tissues, and calicoblastic cells require a supply of calcium ions and carbonate ions for biomineralization and complex macromolecules to form the organic matrix associated with the coral skeleton.
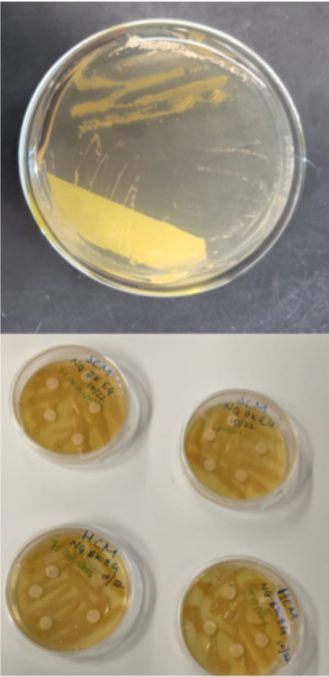
Figure 2: Antimicrobial testing on subcultures from Pocillopora damicornis (Source: Lewinski Lab, VCU)
Separating different cell types and adjusting the growth medium further could enhance survival. Amongst the cell separation methods tested, FACS and a microfluidic device for sorting appear more promising. While a microfluidic device for cell sorting is still being designed and tested by one of our sister teams at the Colorado School of Mines, we tested the separation of symbiotic coral cells from asymbiotic coral cells using FACS successfully (Fig. 4). To ensure separation of all the different cell types though, multiple runs through the FACS machine are still to be tested as are methods of identification of the different cell types.
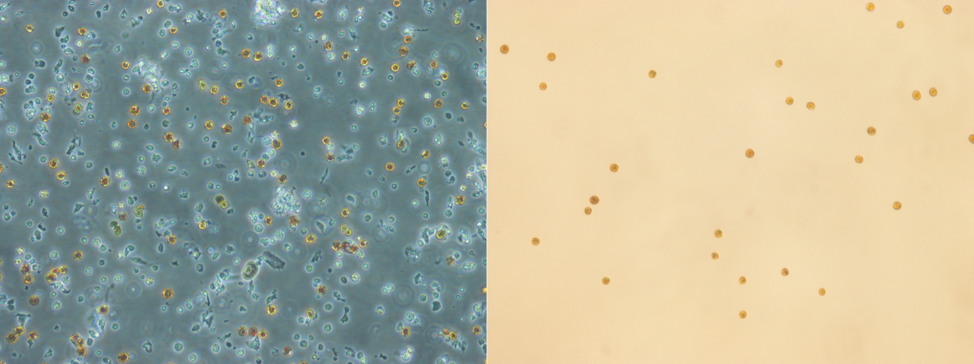
Figure 3: Compound microscope image of harvested coral cells (left) and symbiotic cells separated from other cell types using FACS (right). (Souce: Lewinski Lab, VCU)
How do we grow different cells with such different needs in vitro?
Honestly, there is no short answer. To develop a cost-efficient, long-term successful coral cell culture we need to look into the methods that were developed for cells from other organisms: mammalian cells, algae, plants, bacteria and viruses. The wealth of information and technologies developed in other systems is a great stepping stone but many techniques need to be adapted especially to coral cells. Scientists have successfully grown coral cells before but the life span of these cells was very limited and the duration of the experiments were very short. Nevertheless, these studies provide us with essential information, like cell morphology and size according to cell types, and benchmark measurement for our culture. The maximum coral cells have been kept in vitro is 1 month. The objective here is to extend this to multiple months and more. Having long-term coral cell cultures will allow scientists to test the response of reef-building corals at a new scale to better understand their response to Global Change (using miniature experimental changers). This approach has the added advantage of minimizing the ethical conundrum of repeated testing on ecologically threatened organisms.
In the next Lewinski Team blog post we will discuss designing micro-scale experimental chambers for corals and coral cells. The current scientific advances in biology, fluid mechanics, microscopy and nanotechnologies allow us to design such experimental chambers, or biological reactors, to expose cells to particular conditions and measure their response at the cellular level in combination with live imaging for real-time nano-scale biological resolution. Machine learning and response prediction have been the focus of many studies in recent years, often without considering prediction validation. Coral cell culture combined with mini biological reactors are the perfect tool to validate response predictions, which was not possible previously with such resolution, and push our understanding of these complex organisms. With methods such as bioprinting and 4D cell culture being developed in vertebrate biology, reef-building corals can only benefit from developing coral cell cultures to the stage where they can continue to take advantage of new technological advances to get closer to biological reality in vitro.